This week, a message from Naked Scientists listener Loretta. “I'm curious if your show might cover some of the most interesting case studies of organisms developing tolerance to severe toxins, what genetic insight this gives us about adaptation and natural selection more broadly, and what some of the tradeoffs might be when evolving to have such tolerances.” We're answering her question: how do animals - and even humans - eat poison, and get away with it?
In this episode
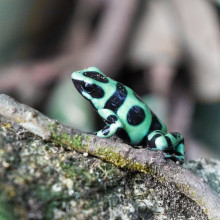
00:45 - Sequestering toxins: the pros and cons
Sequestering toxins: the pros and cons
Hannah Rowland, Max Planck Institute for Chemical Ecology
A toxin is a poison made by a plant or animal’s body. And just as evolution can help these plants or animals develop toxins, it can also help whatever’s eating them to become resistant. But not only that, there’s a biological concept for what happens when an animal eats a toxin, and doesn’t avoid getting hurt: they actually become poisonous themself as a result. It’s called sequestration. Hannah Rowland from the Max Planck Institute for Chemical Ecology took Phil Sansom through the idea by looking at some of the animals who do it…
Hannah - For example, a caterpillar that feeds upon a poisonous plant takes in the chemical defences of the plant, into its stomach, into its gut. And instead of excreting those, they actually move them from their gut into special parts of their body.
Phil - So you don't just survive the poison. You sort of make it your own poison.
Hannah - Exactly that yes. Sequestration is in lots of different animal species. So it's in insects, toads, there are snakes that feed on toads, and then there are even birds called pitohuis that live in Papua New Guinea. Very famously are the poison dart frogs - they also have sequestered chemical defences from the ants that they feed on.
Phil - Are they the tiny brightly colored ones that live in the rainforest?
Hannah - They are. They're in Australia, Costa Rica, Panama, some of which are so poisonous that they are used on poison arrows to kill prey and to even be used maybe in warfare.
Phil - Are all these animals that you're talking about - are they all doing it with the same poison?
Hannah - Some of them they share. So for example, the toads, snakes that live in Japan, and the Monarch butterfly, they have one thing in common - they are sharing the same kind of chemical defence. But they're very diverse. And also most animals don't just have a single chemical defence, but they have a whole suite. There's this battle going on that the plant doesn't want to be eaten, and the caterpillar doesn't want to be eaten by a bird - with a decision that's not really a conscious decision. This really tight specialist relationship means that you get this matching of chemical defences.
Phil - Let's use the caterpillars as a bit more of an example - what's going on inside them? Are there little cells or molecules that are, you know, taking away the poison before it gets into the stomach or what?
Hannah - In some caterpillars that can feed on these plants, but don't sequester the compounds, these chemicals can't pass through the lining of the stomach, into the body of the caterpillar. But in the Monarch, and in other milkweed butterflies that do store these chemical defences, the compounds pass through the lining of the stomach and into the body cavity. This can sometimes happen in a passive way, so it just passes through the lining of the stomach, or it can be actively transported. And when it's actively transported, we sometimes also see that there are special storage units in these insects, special chambers and compartments, where these chemical defences get concentrated and stored, ready to deploy.
Phil - So you've got to not get poisoned. You've got to make the special compartments. You've got to get the poison in there. Is this not quite a lot of effort for the caterpillars or quite a lot of energy that they need to use up?
Hannah - That's a really good question because we always think as scientists taking in chemical defences must be costly to the animal. The costs we would look for are things like, how well do they grow? How quickly do they develop? Maybe, how does their immune system work? We can also think of other costs, like how are they attacked by their predators or by parasites? So we find that lots of animals that sequester pay a really big cost through being parasitised - so by some animals that lay their eggs in a caterpillar, and those eggs then develop within the caterpillar and eat the caterpillar from the inside and then kill the caterpillar and emerge as a parasite. So that's one of the costs that we see. We sometimes see reductions in immune systems. There's also great benefits to sequestering. So some caterpillars grow better because there's sugars in these toxins and they use them to grow. And they certainly defend them against lots of different types of animals that want to eat them.
Phil - Do you mean you can actually see stuff like this if you do comparisons between different butterflies or different plants?
Hannah - Yes. So some research that we've been doing is to look at the costs to Monarch butterflies of feeding on milkweed plants that vary in how much chemical defence is available to the butterfly. We've hypothesised that maybe this is physiologically costly to them. And what we found is that the more that they store as caterpillars and take through into adulthood, the less able they are to create these bright and conspicuous warning signals. So they’re less red and they're less conspicuous. So the really toxic ones just aren't as bright, but they're really nasty.
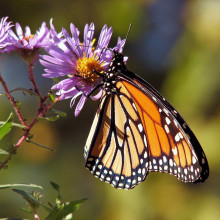
06:35 - Monarch butterflies put poison in their wings
Monarch butterflies put poison in their wings
Anurag Agrawal, Cornell University
While a lot of animals can sequester toxins, it’s done most iconically by monarch butterflies. Their beautiful orange, black, and white wings contain some nasty chemicals. When a bird takes a nip out of a monarch wing, that’s guaranteed to make it ill; the butterflies can survive a damaged wing, and the birds can learn they’re not worth eating. Anurag Agrawal at Cornell University studies this, and Phil Sansom asked him where and how the monarchs pick their poisons...
Anurag - The monarchs are the quintessential example of a butterfly that gets its poisons from its food. The monarch caterpillar feeds on the milkweed leaves and the milkweed leaves are producing poisons called cardiac glycosides
Phil - Cardiac glycosides, you said?
Anurag - Yeah - they bind to a universal animal enzyme and they stop it from functioning, which is what makes them poisonous to most animals.
Phil - What's the enzyme?
Anurag - The enzyme is the sodium-potassium pump and every animal cell, whether it's our human bodies or an insects body, uses this enzyme to shuttle salts across the cell membrane - it's a very critical cellular function. And without that, basically, the cell starts to have either too much salt inside or not enough, which basically causes the system to crash.
Phil - Sounds like a nasty poison!
Anurag - Nasty poison - and very general in the sense that all animals use these pumps so it's going to be very poisonous.
Phil - I just have to ask, you said they were called cardiac though. That means heart, right?
Anurag - Great question yeah. The name cardiac glycoside comes from the fact that these compounds have been used historically in traditional cultures to treat congestive heart failure. There've been several really interesting intersections of societal happenings with cardiac glycosides. And one of them is that Vincent van Gogh, as I assume all the listeners will know, in the last two years of his life and in his paintings, they took a turn. His paintings started having much more yellow, and halos around the lights that are so famous in starry night or in the sunflower paintings. Van Gogh was being treated for epilepsy at the time with extracts of the foxglove plant, which have cardiac glycosides. What we now know is that a side effect of too much of this medicine is yellow vision and seeing halos around bright objects.
Phil - That's amazing. Now I assume the monarch butterflies aren't having their cells unable to take salts in and out, and they're not getting their heart conditions treated. How do they not have all this stuff happen to them?
Anurag - The monarch butterfly has three specific mutations that we're aware of in the genes that code for its sodium potassium pump. Quite remarkably, those three single base pair changes alter the physical structure of that pump, making it about 200 times less likely that a cardiac glycoside will bind and stop that pump from functioning. There probably are other little changes, but there's three main ones that really change the shape of that sodium potassium pump.
Phil - But then the butterflies and the caterpillars go beyond that don't they? Because you said that they actually not only get resistant to the poison, but start to use it themselves.
Phil - Absolutely. Yeah. I think one of the most fascinating things about monarch butterflies is they are themselves poisonous and they advertise it with that highly contrasting orange, black and white coloration. The monarch brings those compounds into its body, packs them away in its wings primarily, and that gets used then as the monarch's defence against predators like birds.
Phil - How sort of physically is it doing that?
Anurag - That's a great question. I'd say we don't have all of the answers. So some of these chemical compounds have chemical attributes that allow them to move throughout the body very quickly crossing membranes. So that would be a passive mechanism of chemical movement into the caterpillars body. The average milkweed plant, however, has, you know, 20 different forms of these cardiac glycosides. And some of them would not be naturally moving passively through the body. And the caterpillars, the monarchs, actively use transporters to move them around to particular places in their body.
Phil - Do you know what genetically is going on in the butterflies that has allowed this to happen?
Anurag - I would say that's one of the big mysteries that we are trying to unravel. From the passive part, it's certainly possible that once an organism becomes tolerant of the compounds, some of them end up in the body accumulating. In experiments we've done with Drosophila the fruit fly we've altered the genes, those same three genetic changes, once they become tolerant of cardiac glycosides small amounts of them already start to accumulate in their bodies.
Phil - You know, it all sounds like a lot of effort to go through when you could just eat a plant that's not poisonous.
Anurag - No question - it's a lot of effort! But one of the consequences is you've got that resource largely to yourself. You know, one of the axioms in nature is that specialisation is beneficial. A jack of all trades is master of none. But we can't really think of it just as the Monarch butterfly deciding to specialise in eating the milkweed. What really more likely happened is that the monarch butterfly ancestor made initial steps towards feeding and specialising on milkweed. In response to that, the milkweed reciprocally evolved a host of defences to try to push the monarchs away. We call that coevolution when two species are going back and forth, evolving in response to each other.
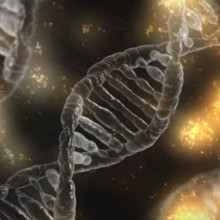
12:42 - Different animals converge on similar toxin resistance
Different animals converge on similar toxin resistance
Anurag Agrawal, Cornell University
In the previous section, Anurag described how there are only a few tiny mutations behind monarch butterflies' resistance to cardiac glycosides. These toxins are ones that a lot of very different animals have independently learned to tolerate - from toads, to bugs, to snakes. And according to Shab Mohammadi from the University of Nebraska, they’ve all stumbled on the same trick...
Shab - The way that all of these animals that are resistant to these compounds known as cardiac glycosides achieve this resistance is a really perfect example of convergent evolution where wildly different unrelated species have all somehow achieved this same adaptive solution. The solution in this case involves mutations in the sodium potassium pump gene. These mutations alter the biochemistry of that region of the protein, so that instead of binding to the toxins, they repel them. They're different mutations, they're different specific changes in the code, but the end product, the end effect is the same and the region in which the mutations occur is the same.
Phil - Is it surprising that all these different, like very different in some cases, animals should have come up with the same solution?
Shab - Yeah, it is. It is very surprising. And it's remarkable when we see cases like this of extreme convergence, where you have this very specific solution to this shared problem.
Phil - But why then are the mutations that each animal uses, why are they slightly different?
Shab - That comes down to the dependence of mutations on the genetic background. We all have the same genes, but each gene has slight differences accumulated over millions of years of evolution. Your gene for the sodium potassium pump protein is slightly different than that of a, for example, hognose snake. And if you make a change to one region that interacts with another region, then they might work together to produce, for example, resistance to a toxin. If one change is there but, then you won't be able to produce the same resistance.
Phil - So how then do you test a mutation in a whole different context, AKA, a whole different animal?
Shab - We genetically engineer a virus to contain instructions to produce a protein with a mutation. And then we infect cells with this virus and the virus infects the cell and instructs the cell to produce this mutated version of the protein.
Phil - In that case, all you're basically getting is this sodium pump protein as if it's from one animal, but it's got the mutation from another?
Shab - Exactly. That's the end product.
Phil - So what do you find?
Shab - Well, we found that these mutations do indeed have highly context dependent effects. Moving the rat mutation onto a snake did not give snake resistance, and moving the mutation from a snake onto an ostrich ended up disabling the function of the protein.
Phil - And, and there was no situation where you could do one better on evolution and make the pump work better?
Shab - Yes, there was actually!
Phil - Oh my God!
Shab - The chinchilla is not a resistant animal, but when you move the rat mutation onto a chinchilla, it suddenly becomes the most resistant sodium potassium pump that I believe has ever been documented.
Phil - What, why?
Shab - We don't really know, but it all boils down to context dependence.
Phil - Now we've also been talking before about the cost of changing such an important protein like this. Is that something that you find in your studies as well?
Shab - There have been a few specific cases where we found other mutations throughout the gene compensating for negative effects caused by the resistance conferring mutations. Then it becomes a question of, well, in what order did these mutations have to happen? And you can imagine that these additional mutations must have been there in place before the resistance conferring mutations could have arisen.
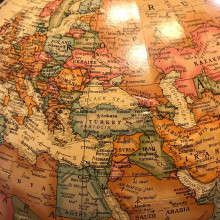
18:31 - Mithridates: the first experimental toxicologist
Mithridates: the first experimental toxicologist
Adrienne Mayor, Stanford University
Humans can become resistant to poisons via the body’s inbuilt adaptability. Eating small amounts of them is sometimes successful; and the technique is called Mithridatism, after a famous figure from classical history called Mithridates who pioneered the technique. Historian Adrienne Mayor described the man to Phil Sansom...
Adrienne - Mithridates was a powerful king of a small kingdom on the black sea. He was born in the second century BC. He was quite a successful rebel against the Roman Republic.
Phil - When did he first start taking poison?
Adrienne - I think he began experimenting even as a young man. When he took over the kingdom, he poisoned his mother and brother, and then he began experimenting himself with poisons and antidotes because he was always in danger of poisoning himself. His mother, even when he was a child, attempted to poison him. Poisoning was pretty rife in that time and place. He experimented on himself and his friends at first, and then when he became a powerful king, on condemned criminals and even allies and enemies. So he goes down in history as the world's first experimental toxicologist.
Phil - Wow. What kind of poisons are we talking about?
Adrienne - Well, there were myriad poisons known in antiquity from toxic plants to snake venoms. There was even poisonous honey in his territory made by bees that fed on toxic plants and then made honey that was a neurotoxin. And there were also many minerals in his kingdom that were highly toxic, such as arsenic.
Phil - Instead of using an antidote, did he actually build up a resistance to them just from taking the poison itself?
Adrienne - Yes. Arsenic was probably the most common poison in those days and it was sometimes called the powder of succession. It's odourless, doesn't have a flavour, but highly toxic. So that would have been the first poison that Mithridates sought to defend himself against because he had used it to poison so many people in his family and other enemies. Now arsenic interferes with metabolism. If you take small doses, however, very tiny doses of it, your liver produces enzymes that will inactivate the arsenic. And if you keep taking these small doses, it allows the liver to produce more of those enzymes that allow you to survive what would normally be a lethal dose. So Mithridates first achieved that. And then in his toxicological experiments with plants and other poisons, he was essentially investigating whether a similar process might work with plant poisons and venoms and other toxic minerals. That's a real scientific method that he was using. And we're talking about the first century BC. So it is quite an incredibly scientific approach to finding an antidote.
Phil - At the same time, it sounds like it's a really dangerous one, right?
Adrienne - Yes. I think it would be dangerous, but he had access to all kinds of resources. It's possible that he even had Hindu treatises on antidotes and poisons. He was able to speak more than a dozen languages. And of course it was risk-taking, but he felt it was worth trying to find a universal antidote. And he finally did come up with a concoction that later became known as the Mithradatium. But Mithridates' invention, you might say, was to not just include beneficial substances, but to also include tiny amounts of poisons or toxins as I said. We do have all of this documented in ancient sources, the problem is we don't know the exact recipe of his concoction that has been lost. On the other hand, physicians of the Roman emperors after the death of Mithridates claimed that they had the recipe of the Mithridatium and every Roman emperor thereafter took a concoction that they believed was the Mithridatium. All royalty throughout Europe took it - I believe up through Elizabeth the first.
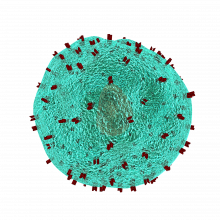
23:59 - Coronavirus: T cells in patients missing antibodies
Coronavirus: T cells in patients missing antibodies
Hans-Gustaf Ljunggren, Karolinska Institute
Our own immune systems follow the pattern outlined by Mithridates. If you expose yourself to a small amount of the toxin, or pathogen, you develop some amount of resistance to it. Adrienne Mayor...#
Adrienne - You can actually stimulate the immune response by taking small amounts of allergens, for instance, that's what doctors recommend to avoid peanut allergies - that small amounts of peanuts are given to children. A similar response works with insect stings, and a variety of different poisons and toxins.
This is crucial during the pandemic, as scientists are trying to understand how good different people’s immune systems are at mounting a resistance to the coronavirus, and whether a successful vaccine is possible. And there’s been evidence for a while now that people who get exposed to low doses of the virus, who haven’t made antibodies to it, have still developed some resistance in the form of immune cells called T cells. That evidence comes from the Karolinska Institute in Sweden, and from researcher Hans-Gustaf Ljunggren…
Hans-Gustaf - T cells are a type of white blood cell. Their function is to recognise virus-infected cells and other types of aberrant cells, and essentially kill them off. Until our paper that had only been very few scattered reports on T cell responses. And we set out to carry out an extensive investigation into the T cell response in the course of COVID-19.
Phil - And what did you find?
Hans-Gustaf - We found that individuals with mild symptoms, or even asymptomatic individuals, that had been living together with infected individuals with symptoms, had developed T cell responses to the virus. Not all of them, but most of them had.
Phil - Were they then immune to the coronavirus?
Hans-Gustaf - That is a difficult question. We don't know. Immunity to us immunologists is kind of relative. They certainly have generated a response towards the virus. If it will fully protect them, we don't know.
Phil - Why had no one noticed this before? Because people made a big deal about antibody tests. Why haven't people been looking into T cells?
Hans-Gustaf - It's fairly easy to develop antibody based assays. It is quite much more complicated to assess for virus specific T cells. It requires, first of all, isolation of cells from patients' blood. It requires specialised laboratories, it requires specialised techniques. While at the same time, you have to take into consideration the fact that we may also have T cell responses to other previous coronavirus infections, some of which may actually partly protect us.
Phil - How do the actual T cell responses though, compared to someone's antibody responses, for example?
Hans-Gustaf - Well, everyone that has mounted an antibody response has also mounted a T cell response. But in addition to that, we identified quite a number of individuals who had not developed antibody responses, but had T cell responses. In fact, when we looked at blood donors in Stockholm, there was a frequency of about 15% of them having antibodies to the viruses. While we found that up to 30% had mounted T cell responses to the virus.
Phil - Was that a surprise to you?
Hans-Gustaf - In part it was a surprise, but at the same time, when you go back to the immunological literature, you may need higher exposure of an antigen, like a virus to mount an antibody response, than a T cell response.
Phil - Can you explain what might be going on in the person's immune system, then?
Hans-Gustaf - The T cells are recognising infected cells as foreign, and the T cells are actually necessary to stimulate another type of cell called B cells. That is the cell that produces antibodies. It might be so that the response has been sufficiently strong to activate T cells, but not strong to stimulate B cells to the degree that they start to generate antibodies, we speculate. It could of course be so that some antibodies orient rating that we cannot detect.
Comments
Add a comment