Regrowing brains, and China's sinking cities
This episode of The Naked Scientists: Scientists give mice a rat’s sense of smell; we find out why some of China’s biggest cities are sinking; and, 14 years after disaster struck in the Gulf of Mexico, might laser-treated cork be able to help us to clean up oil spills…
In this episode
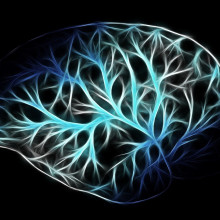
00:58 - Stem cells give anosmic mice a rat sense of smell
Stem cells give anosmic mice a rat sense of smell
Kristin Baldwin, Columbia University Irving Medical Center
In a landmark in brain repair, scientists have used stem cells to regrow a missing part of the mouse brain. Two studies - published in the journal Cell - document how rat stem cells added to embryonic mice engineered to lack the normal smell circuitry, made their way to the brain, turned into the right sorts of nerve cells, and successfully wired themselves in, restoring the missing sense of smell. The study has important implications for how we might go about repairing damaged or diseased human brains in future. Kristin Baldwin is professor of genetics and development at Columbia University Irving Medical Center, and a corresponding author of one of the two papers…
Kristin - Fixing a brain that isn't working is probably the most difficult task that we have to face in medicine. And we would like to be able to understand how to replace parts of the brain that aren't working with other parts that do work. The goal of this study was to test that.
Chris - When I was little I watched a program and Anders Björklund was the guy from Norway and they were doing studies on human patients where they were putting in embryonic tissue to try to help people with Parkinson's disease. Is that the sort of thing you're talking about getting better at? Cell-based therapies, not necessarily with embryonic tissue but with cells that are capable of moving through the brain, turning into new cells to fix things.
Kristin - Exactly. We're trying to find the best way to get cells to go into the brain and actually help it function.
Chris - So how did you do it?
Kristin - We were able to take what are called early embryos in the mouse or blastocysts, which are balls of cells, a couple hundred cells and we can sneak into them about six cells from another species, a rat, that are early stem cells into that. And then they will co-develop in a mouse and become a whole mouse-rat chimaera where the rat cells are peppered throughout the mouse's body. And we can see them either because they cause different fur colours or because we engineer them to glow in the dark, red or green, and the mouse cells do not. So we can look under a microscope and see glow in the dark red rat cells in the brain all over the brain and also in the other tissues.
Chris - And how does this address your question of seeing how we can fix bits of the brain that we want to put cells into in the future?
Kristin - The first question is are there places rat cells can go in the brain and places they cannot? And we were able to clear the whole brain and look through it with a microscope and say basically the rat cells seem to be very good at going almost anywhere in the whole brain. That says this kind of approach has flexibility throughout the whole brain. Great. Now if we wanted to fix a broken brain, we at least think we could do it in a lot of different places. Question two then is, well if there was something wrong with the brain, could the rat cells go in and rescue it and fix it if the mouse's brain had a disease or had been injured?
Chris - And how did you test that?
Kristin - So we tested that by using genetic tricks. We made mouse brains that were missing important cells for the mouse, including those that are important for their sense of smell. So in one case we killed those cells and asked could the rat cells come in and restore that part of the nose? And in other cases, instead of killing them, we just made them very bad at talking to the rest of the brain so that the animal couldn't smell but the cells were still there. And those are sort of similar to things that happen to humans. Either they have a disease where you lose the neurons or you have a disease where they're just not working very well.
Chris - And the question is, you do this to the mouse and then see if the rat cells come in and make good. So before we think about whether they work, do they actually go to the right place and restore what's missing?
Kristin - That was remarkable that they actually go to the right place and the right kind of cells are there. They are adapting to that environment. They're born at the right time and they become the right size and shape to fit into the mouse brain, which is quite a bit smaller than the rat brain. So that is already great news that they can look like they're in the right place and be the right kind of neuron. And that was one of the most exciting early findings we had.
Chris - How did the rat cells know where the problem is in the mouse brain that you've caused and therefore how to fix it and also to look like mouse cells when they're doing it?
Kristin - Right. So all the cells go through the stages of development and they become something called a brain stem cell or neuro stem cell. And at that stage they start to listen to their environment, which tells them depending on where they're sitting, what kind of brain cell, what kind of neuron to become. So if they happen to be in the nose region, they'll become the kind of neurons that are important for smell. If they happen to be in an eye, they might become the kind of neurons that are important for vision.
Chris - So you get the smell system recapitulated, albeit built by rat cells in these mice.
Kristin - Exactly. And that was a wonderful result to see.
Chris - Indeed, because it kind of argues if we did this in a human with human cells, they might work even better. But there should be instruction sets there that will guide the cells to become the right thing. But the key question must be do they work? So if you test the smell system in the mice that have now got a rat sense of smell, do they seem to be smelling?
Kristin - That was the big experiment when we found that being in the right place at the right time could build a rat nose in a mouse. Would that mouse be able to use that nose to do what it likes to do, which is find a hidden cookie. And so we use this test, put a mouse in a cage where a mini Oreo is hidden and ask does it find it? How long does it take? And the mice that had disrupted their smell system wouldn't find the cookie. And when we put the rat cells into a mouse that had no mouse cells that could do this, the mice were able to find a cookie using a rat's nose. On the other hand, this is not just plug and play because if the mouse cells were there but quiet or silent, in that case the mouse couldn't use the rat cells. So we learned already that there are rules to how well your brain can take in different signals or signals for circuits that have been hurt and are now being repaired.
Chris - That sounds like it might be a stumbling block then. So if you have cells that are already there and they're not working properly, they can get in the way and frustrate the repair process.
Kristin - That is one possibility suggested by the work and something that we'd like to investigate further because that's very important to design appropriate cell therapies for humans.
Chris - I have to ask you the question, are there things that mice like the smell of that rats don't? And when you test your mice that <laugh> you find that there are things that they've developed a rat preference for that a mouse never would.
Kristin - Well that's my favourite experiment and we are gearing up to try to do that. It takes a few more tricks, but one thing is that mice actually smell a rat, a cat, or even a snake and are instantly afraid. They'll freeze. Rats are not afraid of rats. So what we'd really like to do is see if that mouse with a rat's nose is no longer afraid of a rat.
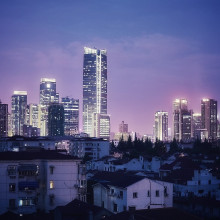
China's cities sinking
Robert Nicholls, University of East Anglia
China is home to around 1.4 billion people - which is more than a sixth of the world’s entire population. The country’s rapid urbanisation in recent years has seen skyscrapers thrown up and groundwater extracted at massive rates to meet people’s needs. But now a new report published in the journal Science has found that this expansion is causing many of China’s biggest cities to literally sink into the ground. Robert Nicholls is director of the Tyndall Centre for Climate Change Research at the University of East Anglia. He authored a commentary on this research…
Robert - A group of Chinese scientists, a very large group of Chinese scientists, studied 82 cities around China, the biggest cities. And they used satellites to measure how fast the land in these cities is sinking or rising. And they found that many of them are sinking sometimes quite rapidly, more than a centimetre per year.
Chris - Oh goodness, that is a lot.
Robert - Yeah, absolutely. I mean, it's a substantial change and when you add it up, it can have quite big sort of consequences. If that continues.
Chris - Why do they think it's happening?
Robert - Several reasons, but probably the biggest one is groundwater withdrawal. These cities are expanding very rapidly and when you have increasing demands for groundwater, you pump it out. And actually the soils beneath these cities, when the geology's right, doesn't happen for every city, you get consolidation. The land literally falls as the water is taken out.
Chris - Lots of cities, though, rely on groundwater for their supplies. Perth, Western Australia has seen its groundwater levels drop considerably. Cape Town in South Africa relies very heavily on it. America, parts of America, has lots and lots of groundwater extraction. So is this likely to be happening everywhere and it's just that the Chinese have spotted it, or is this a uniquely Chinese problem?
Robert - It's not happening everywhere because many areas that contain water underground, like limestone, when you take the water out, they don't drop. I mean, in the south of England, we take water out of chalk and that doesn't consolidate. In the case of China, it's the geology. They've got sands, muds, clays that are geologically recent and they're very old compared to you and I, but they're geologically recent. And when you take the water out, the actual density of the sediment increases. It's called consolidation and that causes the land to sink. But it's not only happening in China. Deltas are particularly prone to this. And there are many, many deltas in Asia, particularly around the Himalayan plateau.
Chris - What's the natural evolution of this then? Does it mean that, because of what you've just said, these buildings will settle eventually and the retreat will stop? Or are they going to continue for the foreseeable to sink like this?
Robert - They won't sink forever. So if you keep on pumping groundwater, eventually all the potential to settle has been used up. But Tokyo, for example, subsided up to five metres in the 20th century. So you can get very large changes. Parts of the Central Valley of California have gone down more than 10 metres, and that's not in an urban area. So you can get very large changes. Why is it damaging? Well, it's not normally uniform. So you find that buildings will literally fall down or in the central area of a city, where maybe the demand for groundwater is highest, will become a bowl and it will flood much more frequently. So it'll mess up the hydrology. And if you are near the coast, if the land sinks, the sea will flood. It is as if the sea level roses, the land sinking, will have the same effect.
Chris - So what can China do about it? Should they be looking for other sources of water? Do they need to be building their buildings differently? What's the solution?
Robert - First of all, it's important to recognise this is happening and then you ask, what can we do about it? One solution is to try and provide other sources of water so that they don't exploit the groundwater. That, however, may not be possible. So the first thing is, can we stop removing groundwater? If not, can we adapt to these changes? But then at least you're not being surprised by them. And back to your point, maybe you can build buildings that won't fall over if they subside or on the coast. Maybe you have to build to keep the sea out or maybe you have to move. I think there are multiple solutions and it will vary from place to place.
Chris - It sounds expensive.
Robert - I think it is costly. Oh, without doubt. I mean, often this problem is seen as a very local problem. And I think one of the arguments we make in our piece is that it's a big problem and as you say, it's costly, but recognising it is going to lower the cost because you're going to start to plan proactively.
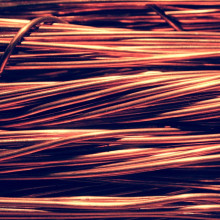
14:25 - Copper solar cell creates "all in one" reaction factory
Copper solar cell creates "all in one" reaction factory
Sam Stranks, University of Cambridge
Researchers at Cambridge University have developed a way to use low-cost copper-based semiconductors to produce photovoltaic - solar - panels that capture the Sun’s energy but then use it to drive chemical reactions on their surfaces. Effectively it’s an all-in-one chemical factory that could produce simple fuels like hydrogen, or even more complex carbon-based molecules. It’s the brainchild of physicist Sam Stranks…
Sam - So generally what we're trying to do is harvest sunlight and convert that sunlight to useful energy to do chemistry. And so here we're splitting water to produce hydrogen, which can be used as a fuel.
Chris - So what is the actual problem you're trying to solve?
Sam - One of them is how efficient we can harvest the sunlight and convert it to energise electrons. Typically this is done with silicon. It's a very good material that can do this very, very efficiently, but silicon's quite expensive to produce. So we've been using copper based materials, which are at the moment very inefficient. We'd be making them more efficient. These are cheap to make and cheap to use.
Chris - If you can pull this off, what sort of improvement do you think you're going to get?
Sam - It's really a step change in what we can do with sunlight. So rather than just thinking about producing electricity, we can start making very interesting materials and making green fuels. So this is for example, carbon based fuels that could be used either in energy applications or in materials developments as well. So this is all done by green solar power.
Chris - And why have you picked on copper?
Sam - Copper is earth abundant, that's a very important thing we want with these sorts of applications. We want to be able to scale them up in a very large way. So we need materials that are readily available and can be easily resourced. Copper is one of those. It's also a very good material that does harvest light quite well and can energise those electrons to do the chemistry that we want to do.
Chris - So talk us through actually how you make it, what you end up with. If you had a physical lump of this in front of us that we could see, what would I be looking at? What does it look like and how does it actually work?
Sam - Yeah, so we make these through a process. It's called electrodeposition. So we use chemicals dissolved in solution that can then be converted to a solid crystal film that will look relatively dark and absorbing because it's absorbing the sunlight quite well. And then we have electrodes on them as well. So we can wire them up and measure the current, for example, that we're getting from these devices
Chris - To all intents and purposes. Then does it work just like a solar panel put on my roof?
Sam - Very much so. It does. So in the same way as a solar cell has an absorbent material that absorbs the light and then the energised electrons are collected as current. We have that in the same way in this material, but the difference is that we have catalysts on the surface that when the electrons reach that catalyst, then they can do some chemistry to be able to reduce water to hydrogen.
Chris - And what was stopping people doing this before then?
Sam - So the concept's been around for a while on copper based materials. They've been limiting because there's defects in these materials where electrons lose their energy, to heat for example. So what we've really done is being able to grow very, very high quality crystals with fewer defects. That means those energised electrons can travel a lot further.
Chris - And is it literally just electricity coming out of wires and then you do something with the electricity or is the step here that you're doing something on the surface of the material? So you're doing sort of two jobs in one, capturing the light, converting it into useful energy, electricity or whatever, and then you're doing chemistry and other exciting things in situ there and then, so fewer losses.
Sam - Exactly. It's integrated on the chip, all in one. So here that's the advantage that we don't have to wire it up and then have a separate electrolyser where the water splitting can happen separately. This is all integrated into one. So it's a very elegant solution and in principle could be very efficient because you've got the absorber itself energising the electrons and then the chemistry is done on the surface of that absorber.
Chris - I'm just trying to visualise that. So would you have it sitting underwater then, or do you have a thin film of water, or have you got little channels so the water or the solution or whatever it is, runs across the surface? How do you do that?
Sam - So it is immersed in water and typically in our cells that we make in the lab, we have essentially a little beaker that it's soaking in. That will be a challenge when we think about scaling it up and there's different solutions for that, but that's for future work.
Chris - So the ultimate goal is it will produce electricity which will split water into hydrogen and oxygen. What, you then tap off the hydrogen and use that? How do you fit this into a sort of production line?
Sam - That's right. So it's extracting out the hydrogen in particular, in this case. And that's something that then that hydrogen could be stored, and it could be used in applications either on site or offsite. So thinking about things like green steel for example, you could use hydrogen to produce that. But there's also some exciting applications beyond just hydrogen. So this is these materials particularly that go beyond silicon in terms of the voltage they can produce. You can start to do interesting chemistry in higher order chemistries. So thinking about making carbon molecules, multi-car molecules as well. And particularly when we can start getting to these higher voltages that materials like copper oxide or other related materials can enable that silicon can't at the moment. So we can start to think about, for example, reducing carbon dioxide to produce carbon molecules. It's really that the holy grail of this solar fuel world is to get to the C2 and above carbon chemistries.
Chris - C2 as in two carbons linked together.
Sam - Exactly right. Yep.
Chris - Is anyone else doing this the way you're trying to do this or have you got something unique here?
Sam - There aren't many others in the world working on the broad field of solar fuels, so it's very much an emerging field. What's exciting about this result is that the photo currents we're producing are going well beyond those results that have been out there so far. In fact, we're moving from something around four milliamps per centimetre squared to something about seven milliamps per centimetre squared under operational current. So we're almost doubling the currents which is really exciting.
Chris - And how does that compare with what silicon can do?
Sam - For silicon, it's more like 20 milliamps per centimetre squared. The problem is there's a compromise in the voltage, so silicon's voltage is much, much lower, whereas we can get much higher. And that's where the exciting new chemistry comes in.
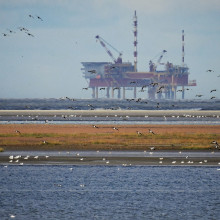
20:31 - 14 years after Deepwater Horizon, can cork treat oil spills?
14 years after Deepwater Horizon, can cork treat oil spills?
Robinson 'Wally' Fulweiler, Boston University & Phillip Broadwith, Chemistry World
It was 14 years ago that the Deepwater Horizon disaster spilled over 130 million gallons of oil into the Gulf of Mexico. It was the largest such spill in history. Since then, scientists have been working on the best way to contain disasters like this. One problem is that chemical dispersants that are often used to break down oil can increase the toxicity in the process. But researchers in Israel and China say they have come up with a clever way around it - which involves using laser-treated cork. To explain more are Chemistry World’s Philip Broadwith and Boston University biologist Robinson - Wally - Fulweiler, who carried out research on the environmental impact of the disaster in the Louisiana wetlands…
Wally - So it was this really intense environment as you would imagine, because there was all of a sudden this huge influx of people trying to work on this problem. We were in and out of boats on salt marshes trying to look for where the oil was coming up on the salt marsh. And so it was really horrifying, to see this like ooky substance <laugh>, for lack of a better word, all over marsh surfaces and beach surfaces and things like that.
Chris - Many people look at the big animals like birds and that's devastating when they get coated. But you were very interested as well in the other things in the environment that often get overlooked. What were you looking at?
Wally - We were really interested in trying to understand how the microbes that live in salt marshes do this process of nitrogen removal. It's an ecosystem service or benefit that salt marshes provide and we were really interested in trying to figure out what could happen when the salt marsh surface was covered with oil and how that would change those microbial dynamics.
Chris - And did it?
Wally - It did. And I think some of the neatest work that's come out of this, if you could say like a silver lining of this awful event, is how much interesting work scientists have done on the microbial communities, that sort of the biodiversity of those microbial communities that are in the environment and how they respond to such an event.
Chris - It was a huge amount of oil that ended up in the sea, wasn't it, Phillip? How did they actually try to deal with it at the time?
Phillip - Yeah, it was huge. It was bigger than any of the sort of spills that we'd seen before and it's coming up from very deep under the water as well. There are various ways that you can try to contain that. You can put physical barriers in the way. But you can also, if you get there in time and under the right conditions, you can apply chemical disbursements. It's a bit like your kind of fairy liquid, it's a surfactant. It's breaking up the oil into smaller droplets and those would be applied at the surface to try and disperse the oil over a bigger area so it can disperse more quickly. But in Deepwater Horizon, they also do this underwater as well and that changes the composition of the oil as it comes up towards the surface, meaning it's in smaller droplets, changing the way some of the different components of the oil go into the atmosphere. So one of the things that was kind of reported later was that there were less volatile things like benzene in the air above the water than there might have been had they not used these disbursements underneath. But the kind of flip side of that is that then the dispersed oil is a mixture of the oil and the disbursements. The disbursements themselves have some toxicity and the mixture itself has different toxicological properties as well. So there can be issues associated with the dispersed oil that are different to if the oil had just been kind of left on its own. It will then spread out over a larger volume of the sea and dispersing the oil doesn't change its chemical composition at all. It's still oil. The only way we kind of eventually get rid of the oil is to have something break it down and that's usually the bacteria. Similarly to what Wally was talking about, there's bacteria in the ocean that will eventually break down the compounds in oil and transport it to the seabed and that kind of thing. So you can contain and collect and pick up a certain amount of the oil, but a lot of it is going to end up in the ocean and it's just going to end up dispersed and over time will be degraded.
Chris - And as Wally was saying, it ended up all over the salt marshes in large amounts didn't it? This paper that's coming out, they say that they've got possibly something else to bring to the party. What are they proposing?
Phillip - It's taking cork, the wood of cork trees, and they are essentially charring it with a laser. It's black, which means it heats up in the sun. That helps to warm it up and make the oil a bit runnier so that it can go into the pores of the material. It's also porous. It also repels water so it can separate the oil from the water and that makes it easier to deal with afterwards.
Chris - Sounds like you'd need a huge amount though, Phillip, and you're back to sort of the problem you were saying where it's still got to go somewhere. So is the idea you come back and scoop the oil soaked cork up later or does it float off and take this toxic cargo onto Wally's salt marshes?
Phillip - My interpretation is that you would have it contained in some kind of matrix material, some kind of blanket. There is a similar material called oleo sponge. It is essentially like a mattress, a polyurethane mattress sponge, that then has a coating of titanium oxide on it, which does the same thing. It makes it attract oil and repel water. And they kind of fashion that into these mats, which you can ring the oil out of. You can put it under the water on the top of the water, it will absorb the oil. You can take that off. You can physically remove the oil by squeezing it out of the pores like a sponge and then you can just go back in and get some more. I would assume that the intention is that this kind of thing is going to work a bit like that. It has the added benefit of this warming in the sun. So if you've got a particularly viscous oil, if it's started to emulsify with the water a bit, if it's heavier oil, some of the lighter fractions have already evaporated off, then you might have problems absorbing it into some kind of material. This kind of thing might help with that. I mean, we're talking about very early stage research. There's a huge difference between doing that and then having a product that will actually be useful in a real life oil spill.
Chris - As we mentioned earlier, Wally, it is 14 years since this all happened. Do we know what the aftermath is now? Has the environment got back together or is there a lasting legacy?
Wally - You know, I've been back to Louisiana. I've been back to some of those areas, but not in a scientific way. Mainly for leisure or conferences or something like that. And so I can't speak directly to what's happening to the places that I looked at, but, from what I know about other places, I would anticipate that most of the visual oily goo is gone, but the residue is probably still hidden and will be for a long time in the sediments as they slowly degrade.

27:56 - Can a random action be replicated?
Can a random action be replicated?
Thanks to Tony Padilla for the answer!
Will - On the show Tipping Point. Often at the end they play out the final three counters as what would've happened. Would the result actually be what would've happened? I argue that such a random action could never be replicated. Good question, David. For those unfamiliar with Tipping Point, it's a game show based around the penny pushing machine in arcades, you chuck a coin in, it drops into an oscillating flat surface and pushes a huge pile of coins ever so slightly further towards dropping off. Even though they never seem to do that. If a contestant decides not to risk the bank, they often run through what would've happened if they had been able to drop another three counters. But would this have actually happened given the sheer number of variables involved? Alan on the forum seems to think so, saying this question concerns a finite number of choices starting from a known assembly. Therefore there is only a finite, albeit very large, number of possible outcomes. A sentiment echoed by theoretical physicist at the University of Nottingham, and author of fantastic numbers and where to find them, Tony Padilla.
Tony - So I think the listener makes a good point, but there are a few things we need to consider. The first is that the descent of the coin is not governed by fundamentally random physics in any kind of quantum mechanical sense, but by classical physics, which is entirely deterministic. So in other words, if you make sure that you drop the coin in the same way from exactly the same position with the same velocity, with the same orientation, the air flow in the room is exactly the same, then indeed the coin will drop exactly as it would've done. Of course, the reality is that you can never guarantee that level of precision. So the coin drop does become a kind of random event. So does that mean we really can't learn anything from Ben Shephard saying, 'well, let's see what would've happened'? Well, not quite, because the most important thing is not the coin drop, but the distribution of the large body of coins at the bottom. Inevitably, the larger it is, the more important it is. So if that body of coins really is piling up close to the edge, then that's what really matters. It's the big body. This is like a game of David versus Goliath, only now Goliath nearly always wins. Of course, what happens to that pile of coins is also a question of probability. It's not that a single coin drop is guaranteed to knock them over, it's just that it becomes more and more probable as things pile up close to the edge. And it's the distribution of that pile of coins that really matters. Incidentally, this pile of coins that you get in arcade games, it does actually behave very much like a fluid with the coins being like the molecules of the fluid and the friction between them giving them a sort of surface tension. And you could do like a cool little experiment where you test some of these ideas. And so what you do is you take a large coin and you drop droplets of water onto the coin one by one. Now, if you've got a pipette, then great use that. If not, just squeeze a wet sponge onto the coin. Now, count how many drops it takes before the tipping point where the water starts to flow off the edge of the coin, and then repeat the experiment multiple times. Now what you'll find is that it's not that the water's going to start to overflow at the same point every time, but it will be more or less the same point. There will be a distribution, and it's kind of the same physics that's governing the penny pusher machine. So by the way, did you know how the arcades make their money with this game? What happens is that at the tipping points, a bunch of coins fall off to the side away from your stash, and this is something that I never knew.
Will - So mechanical probability and arcade advice, we really do it all here. Thank you to David for the question and to Tony Padilla for the answer.
Comments
Add a comment