Cells On The Move
From strange-looking sperm to cancers that spread, we're looking at the light, and dark, sides of cell movement. Plus, a second Covid vaccine approaches the finishing line, but who are the also-rans? We’ll look at who’s most likely to get the vaccine first and whether we’ve got that pecking order right; and how microscopic plants are helping scientists grow replacement human organs...
In this episode
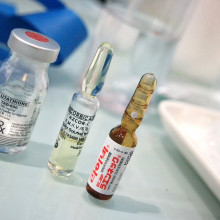
01:04 - COVID vaccines update
COVID vaccines update
Peter Openshaw, Imperial College London
To quote England’s Deputy Chief Medical Officer Jonathan van Tam, "a second penalty has hit the back of the net"; he was referring to the promising results for another Covid-19 vaccine, this one made by the US start-up Moderna. It followed hot on the heels of last week's announcement of similarly encouraging results from rivals Pfizer, who, having since reached the prescribed end-point for their phase 3 clinical trial, are applying to regulators in the US for emergency rapid approval of their vaccine there so it can be rolled out to patients. But to license the agent here in the UK, they also need to get the nod from our regulator, the MHRA. Normally, they would need to go through a trial process in this country too, but there is a special temporary rapid authorisation route known as regulation 174 for use in public health emergencies. MHRA Chief Executive June Raine said this week, "We look forward to receiving the full results of the trials as soon as possible, after which we will rigorously assess the evidence of safety and effectiveness of the vaccine." But what other vaccines are nearing the finishing line, and what does the healthcare profession make of the more than 90% protection rate reported by the two front runners? Chris Smith spoke with Peter Openshaw, an immunologist at Imperial College London...
Peter - It's highly statistically significant. And that's what we're looking for - is statistical significance. It's also much, much better than we expected. We were thinking it might be 50%, 60, 70% if we're really lucky; but nobody was really expecting over 90%.
Chris - The other crucial detail that's now being volunteered by Pfizer, perhaps because Moderna emphasise this point when they gave their results: they have covered a very broad brush stroke of the population when they've done these trials to make sure that their vaccine's relevant to everybody.
Peter - Yes, that's absolutely right. The latest press release is reporting efficacy in adults over 65, and they're also saying that older adults tend to report fewer side effects and milder adverse events.
Chris - Do the side effects look along the lines of what you would expect for a vaccine of any type, and are they within tolerable limits? Because one's always aware of the fact that with this coronavirus, maybe two thirds of people will have no symptoms if they actually catch the virus. And therefore, if you give them a vaccine that gives them any symptoms, you've actually given them a worse outcome than if they just caught it.
Peter - The sort of side effects that are being reported are normal, relatively mild side effects: maybe soreness of the arm, and then maybe a few people feel a little flu-ish for a day or two. I think if we compare that with this situation with catching COVID-19, a high proportion of people are getting really serious disease. So 1% mortality is 10 times worse than getting flu; and we're probably getting at least that number who are getting Long COVID syndrome. That seems also to happen in relatively young people with mild disease. So I've got no doubt at all that the vaccine is much better than catching the disease.
Chris - The one thing we haven't seen data for yet - and this is because no pharmaceutical company has a crystal ball - is what the longer term performance of these vaccines is going to be. They've only asked whether or not people caught it a short while downstream of having been given the vaccine, and that's a bit unhelpful, isn't it? Because we don't really know what will happen in nine months time.
Peter - Or a year, or five years. Yes, absolutely. But then that's unknowable. We inevitably don't know much about how long the antibody response is going to last. The sort of antibody levels that are induced by things like the Moderna vaccine, and also I think by the Pfizer vaccine; it exceeds that that you would expect from natural infection by maybe even a thousand fold. These are really strong antibody responses. So we're waiting to see obviously just how long it will persist and whether the protection is going to last more than one winter season.
Chris - The two vaccines we've heard about so far from Pfizer, BioNTech, and also Moderna - they're genetic vaccines. They've taken the piece of genetic code corresponding to the outer coat of the virus. What other vaccines are currently in trials or being developed? What other sort of irons have we got in the fire here?
Peter - Well, there's some pretty conventional vaccines from companies like Novavax. They've made some of the protein from the virus in insect cells, and then they've packaged it up into what's called nanoparticles. And that looks pretty good too; it's a much more standard approach to making a vaccine, and Sanofi and GSK also have some more regular type of vaccines. The Chinese in particular have been producing a vaccine which is based on inactivated, killed, whole virus. But then we've also got the vaccines which are genetically modified viruses - adenoviruses - which have been taken either from humans or from chimpanzees, and then they slot a gene into that, so that when that virus is injected it then throws out large quantities of the coronavirus spike protein, and the presence of the adenovirus makes the immune system really pep up and produce a lot of immune responses. That's another promising approach, but we don't know how it's going to compare.
Chris - Do you know what the timeline is for these other vaccines?
Peter - Well, the Oxford/AstraZeneca vaccine: we're expecting results anytime soon. I think certainly within the next few weeks everyone's anticipating that the first results may come through. Some of the other vaccines are going to take quite a bit longer, but I wouldn't be surprised if there's two or three that are reported this side of Christmas. The big question then is going to be how long it'll take to gear up and put vaccines into vials and syringes and get them out into arms, and that is no mean feat; that can sometimes take a lot longer than the initial development.
Chris - Do you agree with Sir John Bell, the UK government's vaccine lead, that we're going to be all back to normal by spring? Or do you think he was being a bit optimistic?
Peter - He and I actually are both optimists, but I think in practical terms there will be some groups of people within the population who are going to be vaccinated with the initial first round of vaccines, some of which may actually start to be injected this side of Christmas. But I think in terms of scaling up and being able to reach most of the population, that won't be until spring/early summer.
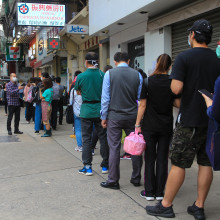
07:53 - COVID vaccine: who's getting it first?
COVID vaccine: who's getting it first?
Philip Clarke, The University of Oxford
Now that vaccines are looking like a realistic prospect, conversations are turning to who should be vaccinated first. The UK's Joint Committee on Vaccination and Immunisation - or JCVI - have published a priority pecking order that’s based chiefly on age. But this was drawn up a while ago, before the second wave arrived, so we wanted to know what factors had been taken into account when this was put together, and whether the strategy is necessarily still the best one. Chris Smith spoke with Philip Clarke, a health economist at Oxford University…
Philip - People who are older tend to be at greater risk of contracting it, and certainly at greater risk of dying. So it makes a lot of sense to use age as a key factor in allocating a vaccine, but there are also other factors one may consider. Why they've gone with age: because they see it as a very simple way to allocate a vaccine. Everyone knows how old they are, but also you can check that when allocating it to individuals in the community.
Chris - And is that broadly what most countries worldwide are doing?
Philip - They're doing some combinations of that. Most countries have recognised all the people in the community should be vaccinated. The age cutoff currently in the UK is at 50; whereas, for example, in a country like France they are only promising to vaccinate above 65 unless you have another health issue.
Chris - What about the fact that we've actually got a mosaic across the country? Some places have got lots of disease activity and lots of older people; some parts of the country have got very low levels of disease activity and a totally different ratio or proportion of older, more vulnerable people. Is that taken into account with these guidelines, or are we just basically planning to treat Cornwall with extremely low levels the same as Manchester with extremely high levels?
Philip - Certainly the guidelines don't distinguish regionally within the United Kingdom. They were written prior to the second wave of the pandemic. And it does seem to make sense to revisit that, because I think what we know from other vaccinations in the context of pandemics is that vaccinating people with the highest risk in the regions where there is the highest transmission is likely to the most good. But this is something where I think the epidemiological modelling could really help inform the trade-offs. Of course there are also logistics of trying to get vaccines across the country, although I think one needs to look at the potential gains from a much more targeted strategy.
Chris - Could part of that targeted strategy also include more emphasis on occupations? Because if you look at data from different parts of the country collected at the peak of the first wave of coronavirus in the UK, there were certain groups that emerged - certain occupational groups, in certain parts of the country - at very high risk. And that doesn't seem to be featuring so much in what the JCVI are proposing.
Philip - That's very true. It's a very clinically orientated view in terms of using age as the primary risk factor, but then also using people who have got various healthcare conditions - such as having diabetes - which also place them at higher risk. And just to give you an idea of the types of risks we're talking about: your risk of dying of COVID effectively doubles about every five years, right? But if you have diabetes, for example, it means you're equivalent in risk to somebody who's probably 5-10 years older. So I think it does make sense potentially to take into account other factors, other conditions; but also the risk of dying of COVID, we very much know, is related to your occupation. There were occupations in the first wave of COVID - for example, taxi drivers in London, and also some people working in the service industry - who had much higher rates than healthcare workers. And so again, I think bringing in those additional factors in addition to the clinical factors will help target the vaccine to where it's likely to do the most good.
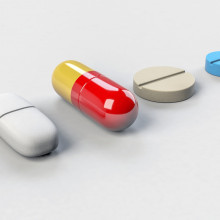
12:37 - Nocebo: statins and side effects
Nocebo: statins and side effects
James Howard, Imperial College London
Have you ever heard of a nocebo? Possibly not, but it’s effectively the opposite of a placebo: you expect to experience side effects when you take a drug, so you do! And a study out this week suggests this nocebo effect could be playing a significant role in the difficulties some people have with taking cholesterol-lowering drugs called statins, as Katie Haylor has been hearing...
James - Most of the time when patients suffer side effects from statins, although they are real, they are probably not caused by the statin molecule themselves, but rather by the act of taking a tablet.
Katie - That's cardiologist James Howard from Imperial College London. James and colleagues have recently published the findings of a clinical trial called SAMSON, which sought to better understand why some people have such a difficult time taking cholesterol lowering drugs called statins.
James - Statins are actually one of the most prescribed medicines in the UK because they can help prevent heart attacks and strokes. But so many of our patients suffer from side effects, and some people have estimated that half of patients have had to stop taking statins within two years of being prescribed to them.
Katie - The team recruited 60 people who'd all actually stopped taking statins in the past due to side effects. These can be wide ranging in severity and type, but in this study, 60% of people had experienced muscle aches and pains.
James - We gave them a suitcase, and inside that suitcase there were twelve jars. And four of those jars contained these statin tablets; four of the jars contained what we call placebos, which are just sugar tablets that look identical to the statins; and four of them were empty. And for every month of the following year, they were told to start a different jar in a random order that we told them. And we also - if they didn't own their own - gave them a smartphone, and they used that to tell us every day, on a scale of 0 to 100, how good or bad they felt.
Katie - Crucially neither the participants nor the scientists knew who was taking what throughout the study.
James - The patients of course knew when they weren't taking tablets, which was the case for four months of the year. But for the other eight months of the year, they didn't know if the tablets they were taking were statin tablets or the sugar tablets.
Katie - And it was tough going for some.
James - 49 of them managed to complete the full year. That means 11 of them didn't. And of those, around half of those had to stop because they found the side effects so bad they didn't want to carry on.
James - Now the 49 that did finish, they also occasionally had to stop their tablets because the side effects were so bad, but the next month they were willing to come back and keep doing the experiment.
Katie - Well the team sat down with the participants and showed them the scores of how they'd been feeling throughout the year and which tablets - statins, sugar pills, or none - they'd been taking.
James - When people don't take tablets, they don't get very many symptoms at all. When they take statins, they actually feel much worse, but they feel 90% as bad taking the sugar tablets. So what we think is going on is that when people take statins, they are concerned that they will feel worse. And actually, this does make them feel worse. And we call this the nocebo effect.
Katie - The team actually found that explaining this nocebo effect to the people on the trial had a pretty big impact.
James - When we sat down with patients and talked about their symptoms and showed them their results, half of them were able to go back on to statins, without problems, and were still taking them six months later. For the half that aren't back on statins yet, a lot of them say they actually want to go back, but because of the COVID crisis and things like that, they haven't been able to make appointments with their GP doctors. So only around half of half of our patients refuse to go back on the statins because they felt they really did have side effects.
Katie - So what about that 10% that wasn't reflected in the placebo group? Are they drug-related side effects?
James - So it's a little bit difficult to say that because whenever you make a measurement in a clinical trial, you know you're not going to be quite precise enough. And because of that, we are saying most side effects seem to be caused by this very interesting nocebo effect. Some patients do get satin side effects caused by the drug themselves because that's the case with all drugs. We're just saying it's probably a lot rarer than we previously thought. By sitting down with patients and showing them the science, you can get them back on tablets that previously they haven't been able to take. So communication is the most important thing.
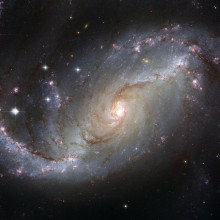
17:52 - Apophis the asteroid edges closer
Apophis the asteroid edges closer
Adam Murphy
On Friday the 13th of November - yes really! - an 11m asteroid skimmed through our outer atmosphere. But astronomers only spotted 2020 VT4 the following day! There was also news out this week that the Sun is pushing another asteroid, called Apophis, closer to us over the next 50 years than we’d like. Adam Murphy has been looking at objects in space like these that come a little too close for comfort...
Adam - Space is big. Really, really big. And it's also, as you might've noticed at night, pretty dark. That means there's a lot of room for things to be hiding out there in the black of space. Some of those things are asteroids and comets, and some of those often get closer to earth than you might think. Objects that get near to earth are called, imaginatively, Near-Earth objects, and they can get close. In 2004 an asteroid about five meters across shot by us, flying below some of our satellites. And if they were to hit, they could cause some real damage. It was an asteroid that killed the dinosaurs after all. And in 2013, a bus-sized object exploded in the skies near Chelyabinsk in Russia. It blew out windows and caused tens of millions of pounds of damages. So it's important to be vigilant.
Many of these objects come from the asteroid belt, which is a belt of asteroids that sits between Mars and Jupiter. Usually, those rocks are content to just sit out there, but sometimes there are big collisions between them and those collisions can send some pretty big pieces of debris our way. Once they've been knocked out of the asteroid belt they continue to orbit the sun, but in long loopy, very oval-shaped orbits. And those orbits can intersect with the Earth's orbit. The gravity of the earth and the sun can even help bring the asteroid back around, like a wasp that refuses to leave you alone on a summer's day. And because space is really dark, we don't always see these things. In 1989 another asteroid call 4581 Asclepius was as close as 700,000 kilometres away, which is about twice the distance between the earth and the moon. But if it had hit, it would have devastated the planet and we didn't detect it until it was nearly on top of us.
One asteroid, in particular, has been on our radar for quite some time and will be ignoring Earth's personal space on several occasions in the next few decades. It's called 99942 Apophis. Supposedly it's called that because the discovers were fans of the Stargate SG-1 TV show, and named it after one of the big baddies. It's certainly how I know the name anyway! It's about the size of the Eiffel tower, and in 2029, will pass under some of our satellites. And it will get close again in 2036, and then again in 2068, although the further into the future you go, the less certain the details are. For a while, this was looking like the most likely contender to hit the planet and cause some damage. At one point, it ranked a 4 on what's called the Torino scale where 1 is "we're fine and there's nothing to worry about", and 10 is "we should prepare for everything to turn into Madmax now."
But there are plans to deal with anything coming our way. The old plan was to just blow it up with a nuclear device but as the general says in one of my favourite movies, Independence Day, that risks turning one dangerous object into many. The new plan is to just give them a push. NASA has a mission planned called the double asteroid redirection test, which will launch in the middle of next year and is going to a pair of asteroids called 65801 Didymos, to test our rock pushing capabilities. Severe asteroid impacts are only predicted to happen once every hundred thousand years, but if we should win the worst galactic lottery and that's going to happen soon well, because I don't know about you, but I wouldn't last too long in a post-apocalyptic wasteland.
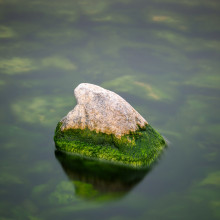
22:19 - Bioprinting algae to grow human organs
Bioprinting algae to grow human organs
Shrike Zhang, Harvard University
Over the past few years, medical scientists have been using 3D printing to create artificial human tissues with the goal of, one day, replacing their natural diseased counterparts. But cells need oxygen, and it’s tough to engineer these ‘bioprinted’ body parts so - that while they’re growing - they don’t die of oxygen starvation or a toxic build up of carbon dioxide before blood vessels can plumb themselves in. So a team in the US are trying to solve the problem using algae. Yes, it does sound strange, but algae are plants: they use light to drive the process of capturing carbon dioxide and releasing oxygen, so by laying down algal cells alongside human cells, one can feed the other and keep both healthy in the process, while an organ develops. Eva Higginbotham heard how it works from Shrike Zhang...
Shrike - We combined our algae and human cells where the oxygen produced by the algae cells can promote the growth of the human cells. After removing the algae we can have a better engineered human tissue.
Eva - What is the problem that you were trying to solve by creating this?
Shrike - Oxygen supply. So providing sufficient oxygen from within engineered tissues has been a major problem or challenge over the years. And if you engineer thicker tissue, it's very hard to get oxygen into the middle of the tissue because oxygen doesn't really go through the thick tissues or dense cells 10. This way that we have devised is to really provide long-term oxygen release from within the engineered tissue. So that's perfectly solving this long-term dilemma that's faced by this whole community.
Eva - What sorts of tissue are people growing in a lab that they need to be able to get oxygen all the way in?
Shrike - This basically applies to pretty much every single type of tissue that people engineer. All the way from, for example, the brain, to the heart, to the liver - there's almost no tissue in the human system that does not have an interconnected network of blood vessels.
Eva - So how have you solved the problem?
Shrike - We use bioprinting to deposit these algae containing structures. If you shine a light across this construct then the algae can actually produce oxygen.
Eva - How on earth do you actually print algae?
Shrike - We use a Jello-like structure containing algae, which are called Bioink. And then if you control how this Bioink is extruded you can start to deposit these algae structures in three dimensions.
Eva - You know what I'm thinking of is whipped cream that comes in a can! You know, where you squeeze things out, either that or like a glue gun?
Shrike - Yeah, sort of yeah.
Eva - And you've actually sent me some pictures that show these algae and it almost looks like you've printed them to look like a honeycomb. Why did you print them in that shape?
Shrike - We printed them in this honeycomb shape just because we were trying to model or mimic human liver structures. But again, using bioprinting, you can essentially deposit any shape or structure that you want.
Eva - And so within these honeycomb circles I can see lots of bubbles, is that bubbles of oxygen?
Shrike - Yes, exactly.
Eva - And so taking this sort of structure that you've made you then throw on some liver cells, is that right?
Shrike - Yeah, basically. So you can throw your liver cells onto the structures. So then these oxygen bubbles that are created by this algae are going to be used to supply the oxygen to the cells.
Eva - Would you then be implanting algae as a part of someone's regrown heart?
Shrike - No. Once the liver tissue is sufficiently oxygenated, you can actually selectively remove these algae patterns from the liver tissue that you can then directly implant into human systems.
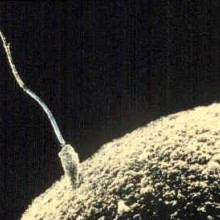
27:24 - Sperm: swimming and surviving
Sperm: swimming and surviving
Allan Pacey, The University of Sheffield
First up in our exploration of cell movement, we're talking about the cells that got us all here: sperm! Allan Pacey is a sperm expert from The University of Sheffield and he filled Chris Smith in on all the details...
Allan - Yeah, a tadpole’s quite a good way to look at most sperm types. There are many variations on the theme. But it's the vehicle if you like that delivers the male set of chromosomes to the female set of chromosomes. The DNA's in the, what might be the tadpoles head, there's then a middle power pack of mitochondria. That's in a thing called the midpiece. And then there's a long tail. Incredibly small human sperm, you'd get 20 of them in a millimeter, if you could hold them down and line them up.
Chris - And that tail is there to provide propulsion. Isn't it? Because one of the key things the sperm has to do is to move.
Allan - Yeah, absolutely, so how does that happen is a good question. So imagine the stick of rock that you might buy at the seaside, chop it in half, it says Blackpool or Brighton through the middle. If you were to chop a sperm tail in half, you would see a series of tubules - they're called microtubules. Those tubules slide together, and that's what gives the tail the kind of whip shape motion that we generally recognise.
Chris - And do sperm do that all the time? Are they constantly swimming even when they're being made in the testicles, for example, or can they turn that on and off?
Allan - So before sperm are released they're not swimming. They're being stored. But when they're released, it's the removal of the chemistry of the male body and it's the stimulatory chemistry of the female body that switches them on. Now, sperm will swim until they die pretty much. In human terms, in higher mammals, sperm often swim close to the egg, and then they tend to power down and they wait for the egg. It's a really elegant kind of holding mechanism if you like.
Chris - Like go an enormous distance, don't they? Because from the point of entry into the female reproductive tract, in a human, they've got to get all the way up through the uterus. And then to the top part of the oviduct, the channel that carries eggs from the ovary, where it's come from in order to meet the egg. When they actually meet the egg, how do they know they've met the egg and how do they actually then do the business of fertilising it?
Allan - Well, actually sperm get quite a helping hand from the female's body to get to the site of fertilisation. So there's muscular contractions of her body that help the sperm get there. And then once they get into that oviduct, there's temperature differences within the oviduct that cause the sperm to move towards the egg. It's slightly warmer towards the egg end. There's a chemical signal that helps them get there. We're not quite sure what that is, but there are chemicals that are released from the egg or the cells around the egg that draw the sperm in. And then there's also fluid movements that push the sperm along towards the egg. A man, a human male will ejaculate 50 or 60 million sperm into a woman. By the time you get to the oviduct, there's probably only around half a dozen.
Chris - Well, if just a handful make it, does that mean then that basically a massive selection process has happened? All those sperm that started the race - it is literally survival of the fittest. It's the best sperm that makes it?
Allan - To some extent that's right. It is a big race, but it's very highly coordinated. So the point that ejaculation happens, then it's only nicely shaped sperm that enter the cervix. If the sperm are malformed - got two tails, two heads, or they just that bit slightly differently made, then they're not going to get through that cervical mucus. It's very thick. You need to be kind of aerodynamically and fluid mechanically very sleek to get through that. In the uterus sperm are under attack from white blood cells. So, you know, you've got to get to the fallopian tubes pretty quickly if you're going to avoid death by leukocytes. And then when they sense these signals from the egg, those sperm then change the way that they swim. They start to hyper activate -we call them the tailies - beating around and providing great force and energy to help that final winning sperm get through the outer coat of the egg. It's probably only at the last few millimeters of sperm getting towards the egg that there's actually a sperm by sperm kind of race if you like, but there's probably only about half a dozen sperm at that point in the race.
Chris - Yes. It's a miracle that any of us are here really, when you think about it, isn't it? But you brought up the question of sperm shape. Now a few years back, I was in Norway and I went to visit a gentleman there who's a biologist who works on bird sperm. And I was very surprised when he told me that if you look at birds that are very promiscuous and they basically they'll go with anything that's willing, they have very different shaped sperm, he said, to birds that are highly monogamous, like swans, for example, they mate for life. What's he getting at?
Allan - So promiscuity within a species does impose some sperm-specific selection pressures. So if you're a male in a species where there's lots of competition for females, you better make sure your sperm is the best. And that evolutionary pressure means that across the animal kingdom, there's been some quite elegant solutions to the sperm problem and quite subtle refinements that might give males a competitive edge. Within primates, there's a classic example. So chimps, highly promiscuous, they have big testicles and the sperm they produce swim really fast and they're very sleek and elegant. Gorillas, not particularly promiscuous. And they by comparison have pretty terrible sperm. Humans? Kind of in the middle. We've got a reasonable number of pretty well sperm, nicely made sperm. Um, but we've also got a lot of badly made sperm too. So depending on where you are on this kind of promiscuity scale, it does have a huge impact on what the sperm look like and how well they're made.
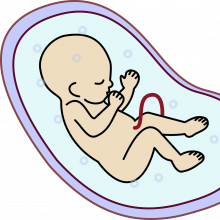
34:30 - Neural crest cells: crawling around the embryo
Neural crest cells: crawling around the embryo
Paul Kulesa, Stowers Institute for Medical Research
Every single one of us exists because a sperm successfully fertilised an egg. But the resulting embryo faces a problem: how to ensure that the trillions of cells that it gives rise to - and which make up the body - end up in the right places. One group of cells is called the neural crest. They form down the length of the embryo above what’s going to become the spinal cord. But from there, they have to migrate around to the front of the embryo - passing through lots of other developing tissues on the way - to give rise to a host of different structures including the nerves supplying the skin, melanocytes that give us our protection from the sun, and they even form the face. These feats of navigation mean the neural crest can teach us a huge amount about how cells move, and Paul Kulesa, from the Stowers Institute for medical research, studies how they do it, and what can sometimes go wrong, as he told Eva Higginbotham...
Paul - Neural crest cells are a multipotent cell type. That means they can become a number of different types of cells that include neurons and bone and cartilage and pigment cells. And they contribute to nearly every organ. But the problem is that neural crest cells must travel long distances to reach peripheral targets, where they contribute to organ assembly. They encounter complex developing tissues during migration in an embryo that's growing and undergoing tremendous amounts of dynamic movements of the underlying tissues through which they're crawling in the fibers. So it's a very dynamic microenvironment and it's as if they're crawling through a very dense like Amazon jungle, but the jungle is dynamic and moving and growing plants as they're trying to get through the jungle.
Eva - So these cells are sort of crawling through this complicated surface of other cells and other things that are going on. How do they actually move themselves forward?
Paul - Well, we know from cell culture studies that cells will extend a protrusion and then anchor that protrusion and then degrade whatever fibers are in their way and attach to something that they can hold on to, and then pick up the attachments behind at the backend of the cell and then pull themselves forward.
Eva - Is that kind of like how you kind of think of a slug moving or a snail, they kind of push out some part of themselves and then drag themselves along?
Paul - Right, right. Very much so because you can see in vivo, when you're imaging, you can see bits and pieces of the cell membrane that are left behind actually, as the cells are crawling through. And so it's almost going back to the analogy of the jungle as if they're going through very dense thorny bushes and shrubs and getting pieces of clothing ripped off on the thorny bushes as you go. And it's very much so it seems like with the cells.
Eva - How do they manage to actually force a protrusion out? Cause if they're moving through this really dense forest, how do they get enough sort of energy and force behind them to push a protrusion between cells to allow them to sort of dig their way through?
Paul - Right. Well, there's a couple of different ways that we know of that cells can do this. They can start to initiate a protrusion of the membrane by bringing in machinery of the cell, which builds fibers in the cell. But there's also another way that a cell can initiate a protrusion and that's by opening water channels, aquaporins, within the cell membrane. So Aqua for water and pour for porins. And having water do the job to initiate the protrusion.
Eva - Like creating holes almost that allow the bit of the membrane that they want to force its way through, to kind of inflate?
Paul - Right. It's as if in the dense jungle, there's only small gaps, then you can stick your arm through there and then cut away at the fibers in order for you to then move more of your body through that dense jungle.
Eva - What happens if something goes wrong in that process?
Paul - Ah, well, if they can't make it to their target in the head, then the cranial facial pattern can be disrupted because they give rise to bone and cartilage of the face. And so two of the more common human birth defects are cleft lip or cleft palate, or problems with the nerves that innervate your jaw. And neural crest cells contribute to the heart. And so you can have congenital birth defects to the heart flow tracks. One of the major early pediatric cancers is neuroblastoma. It is a solid tumor that forms outside of the brain. And it's very common in infants that are zero to five years old. And what happens is the neural crest cells that are contributing to the peripheral nervous system and becoming neurons and having to wire a neural circuit have some malfunction and that can lead to pediatric neuroblastoma. We're looking to see whether aquaporins and other invasion genes that are typical of the embryonic neural crest relate at all to predicting the potential of a neuroblastoma as a solid tumor to become what's called metastatic or to leave the primary tumor site and invade other tissues.
Eva - So are these aquaporins prevalent in other cancers that we know are likely to metastasize?
Paul - Yes, well, there's been some work in glioblastoma recently. That's compared patient samples of the tumor core versus infiltrating cells. And we know that aquaporin-1 is expressed by the infiltrating cells. So certainly in glioblastoma and in other invasive cancers. So it may be that it's both predictive of metastatic potential and a clinical target.
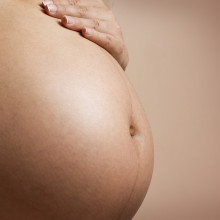
41:20 - Microchimerism: when foetal cells escape
Microchimerism: when foetal cells escape
Kiarash Khosrotehrani, The University of Queensland
Microchimerism occurs when foetal cells cross the placenta and travel through the mother’s body, sometimes taking up residence in her tissues and even her brain. It might be one way that a foetus tries to help repair some of the damage that pregnancy can do to a woman’s body. At the same time, because these foetal cells can persist in a mother’s body for decades afterwards, it might also explain the surprising surge in autoimmune diseases seen in women who have had children. Eva Higginbotham spoke to Kiarash Khosrotehrani, who is at the University of Queensland...
Kiarash - Microchimerism is something that happens during pregnancy where a very few cells from the baby crossover into the maternal blood, and then go to the maternal circulation and then stick in different tissues.
Eva - And what kind of cells from the foetus are we talking about? Are these, you know, differentiated cells like skin cells, or are they more like stem cells?
Kiarash - There is a bit of randomness in everything that's being transferred. However, as a lot of the cells in the foetus are primitive, there's a large proportion of them that are stem cells and that's what makes them survive long-term and persist for decades in the maternal body.
Eva - That's a really long time! Where are they hanging out?
Kiarash - That's an excellent question. We actually don't know for sure. Where we have found them so far is for example, in the maternal bone marrow. So we can find in the maternal bone marrow, stem cells that have been there for almost four or five decades, or we can find them also in other tissue, where we find them the most, when the mother has some sort of injury, because these foetal STEM cells tend to home towards that injury in the mother and try to fix it, try to repair it, as would the natural STEM cells. So that's where we find the most. And we have been finding these foetal cells in a variety of tissues, in the liver, in the skin, in the thyroid, and so on. But we think that naturally there resides a lot in the bone marrow.
Eva - How do they get by, doing that without being rejected by the mother's body? Because you'd think that the mother's immune system would recognise these cells as non-self and therefore try to get rid of them.
Kiarash - Absolutely. So, we think that at the initial stage, when the transfer happens during pregnancy, these cells benefit from the exact same mechanisms that the mother has in place not to reject the foetus. So during all pregnancy, actually the maternal immune system is sort of dampened, and reduced so that it doesn't react against the foetus, which is something that should be recognised as foreign. So these cells are undergoing the same principles, and then later on, because there are few of them and probably because they're hiding in different places in the maternal body, and don't cause any trouble, they essentially don't generate any danger signal for the maternal immune system. And they're somehow being ignored.
Eva - How do these cells actually cross the placenta? Why are they going across? And also, is this just a normal thing that happens in every pregnancy? Just like a thing that happens, or is it a pathological weird thing?
Kiarash - So this is something that happens in every pregnancy, in really a large set of studies. We can identify these cells in the maternal circulation, if we look hard enough. The reason why they're crossing, we believe are small ruptures of that placental barrier. So between the mother and the foetus, there's always a barrier. And we believe that every now and then, because of movement, because of very small events, this barrier breaks down and a few cells from the foetus can be transferred into the mother, or vice versa, before the barrier is reestablished. In scenarios where this barrier is actually much more disrupted, if the mother, for example, has high blood pressure, if there is any placental abnormality, this transfer happens actually much more frequently and we can find many more foetal cells in the maternal circulation.
Eva - So how many cells are we talking, in a sort of normal pregnancy?
Kiarash - The reason why this was called microchimerism is because of these proportions of one in a million, to one in a billion. So they're quite rare cells. And then when you're talking about what proportion of these are actual stem cells, the rates are even smaller. So, you know, there would be almost one to two cells per litre of maternal blood, that you'd give rise to actually, a whole stem cell population. So they're quite rare.
Eva - What if a mother had multiple children, would that mean that it's likely that she's not just a chimera with one child, but actually with as many kids as she's had pregnancies of?
Kiarash - That's absolutely correct. And there are really strange interactions in terms of how one child influences the microchimerism from the second child, and the third child, there are some groups that have been actually studying that.
Eva - So like sibling cells hanging out in the mother's body, are kind of fighting it out in some way?
Kiarash - Or one immunising the mom against the other, or yeah, there is this type of interaction, exactly.
Eva - Wow. Okay. So it starts early! So can these cells cause any harm in the woman's body?
Kiarash - So there was a whole range of studies, trying to see whether these cells could actually trigger the maternal immune system to react against different tissues, and be maybe responsible for autoimmunity. There's been a number of studies that looked at, for example, the presence of foetal cells in mothers with scleroderma, or systemic sclerosis, which is essentially an autoimmune disease, where the mother's immune system reacts against itself. So these studies have been conducted. There are associations, but they've never been able to show causality, which means that we don't know if the foetal cells are the cause of the autoimmune disease, or they're a consequence of the autoimmune disease homing to the injured tissues to actually try to repair.
Eva - The other pathological thing that I was thinking of is tumours. If you have all these stem cells that are sort of lodging themselves in various locations in the body, do we know if these foetal cells can be responsible for tumours in the mother's body?
Kiarash - As far as it's been studied, we have never found really, malignant tumours being entirely formed of these foetal cells. There have been reports of some benign tissue, especially in the thyroid, where the foetal cells have formed part of it, like benign adenomas, but not really anything that's been malignant and causing metastasis, and so on.
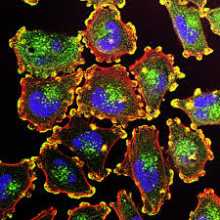
48:08 - Cancer metastasis: why does cancer spread?
Cancer metastasis: why does cancer spread?
Sakari Vanharanta, MRC Cancer Unit and Cancer Research UK Cambridge Centre
Cancer metastais occurs when rogue cancer cells break away from a tumour and spread, often via the bloodstream or the lymphatic system to other parts of the body. But why do cancer cells but not healthy cells do this, and what determines where these cells tend to spread to? Sakari Vanharanta is a cancer biologist at the MRC Cancer Unit in Cambridge and Cancer Research UK Cambridge Centre, and his lab studies this process, as he told Chris Smith...
Sakari - I think they don't necessarily want to spread, but cancer is a disease of uncontrolled growth. And these tumour cells divide and divide. And there's so many of them that they end up just spilling out of the primary tumour and ending up in different organs. And then some of those cells eventually survive there, and form a secondary tumour. So I don't think this is an orderly process in cancer. They just move randomly and end up in secondary organs because of that.
Chris - But some cancer types do seem to "prefer" in inverted commas, to spread to certain parts of the body, and certain other organs don't they?
Sakari - Absolutely, there is organ specificity to the metastatic process. And this is something that is kind of poorly understood still, but it seems that there's a match between a certain cancer types and a certain secondary sites and cancer cells when they end up at there, or if they end up there, they have a higher chance of surviving, and that's why there's this predominance of certain types of metastatic sites.
Chris - Is it just the cancer cells, the abnormal cells that have made the primary tumour, that then go off and spread, or do they have the potential to subvert and manipulate other healthy cells in other places, to make their spread and invasion into other sites in the body, more likely?
Sakari - Cancers are more like ecosystems, there's multiple different cell types there. And cancer cells recruit various types of normal cells, and they exploit those, and they do this for many purposes, including migration and for the purpose of leaving. So some cancer cells can follow, or tag along with other normal cells, and that will help them move. So absolutely it's a process in which cancers very much exploit normal cells.
Chris - We were hearing earlier about the amazing journey that these neural crest cells take when they're migrating from where they're formed, in a developing embryo, to end up all over the body and giving rise to all kinds of important structures, presumably since cancer cells have access to all of the same genetic programs that a developing embryo would have, can they just turn on those developmental programs and basically pretend as though they're back in an embryo, and go wherever they want, is that part of the reason why they're so destructive?
Sakari - So in cancer, many of these developmental programs are activated in the wrong place. And I think an example of this would be melanomas, which develop from the melanocytes that were just discussed in the context of neural crests. So the normal function of the developing neural crest, is to migrate through the developing embryo. Now melanocytes don't normally do that, but the cancer causing mutations may allow them to activate the same programs. And that's one of the reasons melanomas can form metastases in many secondary sites.
Chris - And is there any evidence that cancers, when they're in situ, and they've got these distant metastases, these deposits in other parts of the body, are those cancers in cahoots? Do they talk to each other? Is there a sort of chemical conversation going on between tumour deposits around the body?
Sakari - Between the different metastatic sites I think it is difficult to measure whether those sites communicate, but cells travel from one metastatic site to the next. So through these kind of messengers, they would be exchanging information.
Chris - So you're saying a cancer can not only spawn a new tumour in a new place, but sometimes cancer cells will come out of one metastatic deposit and go to another one?
Sakari - Absolutely. So there's genetic evidence showing that again, like an ecosystem of different tumours in different parts of the body, and there's clones that move from one place to another, and then start growing there.
Chris - And does that affect the way that the tumours grow? If one of them discovers a really good way to grow, can it take that information or that know-how to another tumour site, and stimulate that to grow more?
Sakari - Yes. For example, if you have cancers under therapy, you could have resistant clones that emerge in one metastatic site, and then if it's migratory, it could colonise new sites or preexisting metastatic sites, and then help them grow as well.
Chris - Oh goodness. So that would explain why, when you have advanced disease, it can suddenly accelerate uniformly. So it's not just one bit of it that discovers how to grow better. The whole lot seems to progress, and progress increasingly quickly as the disease advances.
Sakari - Very true. So metastatic tumours can behave exactly like that, when you get resistance, it can happen in multiple places simultaneously, but it can also happen independently. So they could have mutations that target the same gene, independent mutations in different sites. And that could also lead to the same outcome.
Chris - But now that we know all these things, and we understand a lot more about the processes that lead up to cancers beginning to spread, the fact they get into tissues and establish metastases. How does an understanding of that mechanism inform how we treat patients?
Sakari - I think if we understand how metastatic sites, for example grow, of course it can help us develop targeted therapies for those very specific processes. And there are examples, like anti-oestrogen therapy in breast cancer. We know that breast cancer cells like oestrogen, so to prevent metastasis, we give patients anti-oestrogens to prevent that from happening.
Chris - And are there any specific things we've noticed that do by and large repeatedly lead to cells wanting to go walkabout? And by interrupting that, we have a chance to interrupt across the board, the spread of cancer? So we would turn a cancer from something that's highly progressive and virulent and nasty, into just a chronic indolent thing that we live with, a bit like high blood pressure, I suppose.
Sakari - I think that's the hope, but I don't think we are there yet, because we don't understand the process well enough. I think to inhibit the initial spread of cancer is very difficult because it's difficult to find the time window of applying such a therapy, but trying to prevent already disseminated cells from forming clinically meaningful metastases, I think that's why we should try to go.
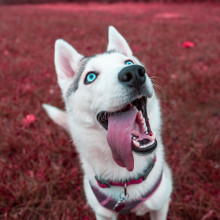
54:58 - QotW: Can loud music hurt my dog?
QotW: Can loud music hurt my dog?
Adam Murphy put the question to animal behaviour specialise Nancy Dreschel...
Robin - I often wonder when I listen to music in the car when my dog is with me: since they hear higher frequencies than humans, do they also perceive for example loud music louder than us?
Adam - Nothing better than rocking out on a road trip. Dog Days are Over, Hungry like the Wolf, How Much is that Doggy in the Window... so many choices. But it could be hurting your furry friend? Nancy Dreschel is a specialist in Animal Behaviour from Penn State University.
Nancy - Noise induced hearing loss in dogs occurs and is related to the decibel level of the noise, rather than the frequency. The most common cause of noise induced hearing loss in humans and dogs is injury to the hair cells inside the inner ear. This can occur after exposure to loud noises like that stereo turned up too loud, or even a kennel or shelter where there are a lot of barking dogs.
Adam - So same as us in a lot of ways, I hate that feeling in your ears when a noise is too loud. And some dogs have bigger ears than I do.
Nancy - Researchers at the University of Illinois described hearing loss in working dogs. It is thought that a one-time exposure to a really loud, percussive noise, like a gunshot over the head of a dog while hunting or loud sirens can cause both temporary and permanent hearing loss. So, your dog is as susceptible to hearing loss related to these activities as you are.
Adam - Dogs are susceptible then, but how does that translate to rocking out in the car with your four legged friend.
Nancy - I think another question is, does your dog enjoy the same music you do? While you can tell your sister to change the radio station if you aren’t enjoying her music, your dog can’t tell you that he doesn’t like your selection. Watching your dog for signs of distress like licking his lips, putting his ears back, panting, or yawning while listening to music, may give you a clue that your dog doesn’t share your musical tastes. On the other hand, if you and your dog are having a good time, and he seems happy with you singing along with the radio at a reasonable level, enjoy your time together and rock on.
Adam - Thanks Nancy for making sure we’re not barking up the wrong tree. Next week we’re looking for an answer to this question from Robert.
Robert - Hi. I’ve been wondering, in terms of net greenhouse warming effect, which is better, sending your organics to a landfill, or composting them at home?
Comments
Add a comment